Transoral robotic surgery for oropharyngeal squamous cell carcinoma: a scoping review of novel techniques in margin assessment
Introduction
Oropharyngeal cancer remains the sixth most common cancer worldwide (1) with almost 100,000 cases in 2020 (2). We are in the midst of an epidemic of human papilloma virus (HPV)-related oropharyngeal cancer. Temporally coinciding with this was the introduction of transoral robotic surgery (TORS) in 2005. The combination of an emerging treatment-sensitive disease and a new surgical technology has changed the landscape of treatment in these patients. TORS is now an established approach for resection of oropharyngeal squamous cell carcinomas (OPSCC) with acceptable postoperative swallowing outcomes and quality of life for patients (3). It provides minimally invasive access without major tissue disruption through a natural orifice and caters for a three-dimensional view with enhanced freedom in manipulation of surgical instruments.
Despite significant advances in technology, the lack of haptic feedback and proximity of tumour to critical neurovascular structures proves a significant challenge in achieving negative margin resections. Literature reports that microscopically positive and “close” margins carry a significantly increased risk of local recurrence with poorer prognostic outcomes (4). Currently, the Royal Society of Pathology defines a surgical margin of more than 5 mm from the invasive tumour to the resected margin as clear and a 1–5 mm as close (5), concurrent with the EORTC trial (6). These close and positive margins are said to range between 0–20% (7) which is far from the accepted institutional values of 0–5%. Given the importance of positive and close surgical margins in the prognosis of patients, these have significant implications and are typical indications in deciding the role of adjuvant chemoradiotherapy. EORTC trial (6) has highlighted the significantly improved 5-year progression-free survival, locoregional control and overall survival in patients undergoing adjuvant chemoradiation post-surgical therapy. Hence, the evidence recommends chemoradiotherapy to those with positive margins or other high-risk features (8). Thus, contemporarily there is imperfect tumour margin assessment in TORS patients which needs addressing to allow accurate assessment of the need for adjuvant chemoradiotherapy in these patients.
Currently, frozen sectional analysis is the most commonly employed tool in surgical oncology for margin assessment. It remains a time-consuming approach with the potential for discrepancies to occur when the final formalin pathology is available. Hence, this review sets out to scope innovative intraoperative margin assessment techniques that have been used to aid TORS in achieving negative margin resections in patients with OPSCC. It will aim to document the way these techniques work, results of the techniques on margin resections, limitations within technique and their scope of introduction to common clinical practice. We present this article in accordance with the Preferred Reporting Items for Systematic reviews and Meta-Analyses Extension for Scoping Reviews (PRISMA-ScR) reporting checklist (available at https://www.theajo.com/article/view/10.21037/ajo-24-9/rc).
Review question
What are the margin delineation strategies in patients undergoing TORS for OPSCC?
Inclusion criteria
Context
This scoping review considered studies that included patient population of male and female adults (aged 18 years or older) who have undergone TORS for OPSCC. Studies with other subsites of cancers were only included if subset data of OPSCC was provided. Studies that evaluated TORS with or without other adjuvant modalities including chemoradiotherapy were included. Studies focussing on other modalities of transoral surgery including but not limited to transoral laser microsurgery were excluded. There were no limitations placed on geographic location, gender or specific racial contexts.
Study designs
The review considered all study designs including experimental study designs, analytical observational studies and descriptive observational studies. Systematic reviews and meta-analysis were not included. No further limitations were placed on the dates of publication and all countries/health systems were considered.
Outcomes
This scoping review only considered studies with surgical margins as their primary outcome. The surgical margin status had to be correlated to a specific margin delineation strategy and data without this specific correlation was excluded.
Methods
This scoping review was conducted in accordance with JBI methodology for scoping reviews (9). Ethical approval was not required given the nature of the scoping review. Findings were reported in accordance with the PRISMA-ScR checklist (10). This review was conducted without a priori protocol.
The search strategy aimed to locate published studies in English from the inception of included databases: MEDLINE (PubMed), Embase and CINAHL. An initial preliminary search of MEDLINE found keywords in the areas of interest by analysing titles, abstracts and index terms of relevant studies. Keywords in each search strategy included “Margins”, “Surgical margins”, “Transoral robotic surgery”, “Oropharynx” and “Squamous cell carcinoma”. Further synonyms and relevant keywords were identified and were used to develop a comprehensive search strategy of these databases (Table S1). Each search strategy was amended to suit the different codings of each database. Once a search was finalised, all identified citations were collated in Endnote 20 (Clarivate Analytics, PA, USA) and duplicates were removed. A reference list was extracted and uploaded into Covidence 2023 (Veritas Health Innovation, Melbourne, Australia). Following a pilot, two independent review authors (S.G., G.K.) screened titles and abstracts, then followed by the full text articles for inclusion in this scoping review against the inclusion and exclusion criteria. Disagreements were resolved through discussion and consensus with the consultation of a third author. The reference lists of all included articles were screened for additional studies.
Eligible studies underwent a risk of bias assessment by two independent reviewers (S.G., G.K.) for methodological quality using JBI critical appraisal tools as appropriate for each study design with results attached (Tables S2-S5). All studies regardless of the results of methodological quality underwent data extraction. Data extraction was piloted and extracted using an independently formed tool. Data extracted included study design, year published, country published, sample size and their key findings. No assumptions were made during data extraction.
Results
The search identified a total of 364 studies in English with 286 remaining after duplicates were removed (Figure 1). Titles and abstracts were then reviewed against the inclusion and exclusion criteria yielding a total of 53 articles to be analysed in full text. All 53 articles were able to be retrieved in full text form. Of these articles, 9 were included in the final study.
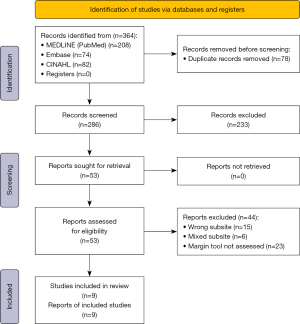
Of the 9 studies incorporated (Table 1), 2 focussed on narrow band imaging (NBI) and 3 on fluorescence lifetime imaging (FLIm). The remaining studies were singular on topics including frozen section analysis (FSA), intraoperative ultrasonography (IUS), near infra-red light (NIR) and high-resolution fibre optic microendoscope. Studies included were published between the years of 2014–2022 given the recent advance and increased adaptation of TORs procedures in head and neck units around the world.
Table 1
Margin delineation tool | Title | Author | Year | Country | Study design | Sample size of OPSCC patients | Key findings |
---|---|---|---|---|---|---|---|
Frozen sectional analysis | Association of Intraoperative Frozen Section Controls With Improved Margin Assessment During Transoral Robotic Surgery for Human Papillomavirus-Positive Oropharyngeal Squamous Cell Carcinoma | Yu et al. | 2022 | USA | Cohort study | 170 | Frozen section analysis had an accuracy of 94.1% with a sensitivity of 85.1% and specificity of 97.4% (n=85). Sensitivity increased to 88.9% with a statistically significant difference of 6.1% (95% CI: 3.9–8.3%) with an intraoperative control biopsy |
US | Intraoperative Ultrasonography During Transoral Robotic Surgery | Clayburgh et al. | 2016 | USA | Case series | 4 | Able to identify the margins of tumour separating abnormal and healthy tissue as an echogenic line. US tumour depth measurement accurate to 1–2 mm |
NBI | A novel approach emphasising intra-operative superficial margin enhancement of head-neck tumours with narrow-band imaging in transoral robotic surgery | Vicini et al. | 2015 | Italy | Prospective non randomised cohort trial | 44 | Sensitivity and specificity of NBI was 72.5% and 66.7% respectively. NBI group had a higher rate of negative lateral margins when compared to WLI (87.9% vs. 57.9%, P=0.02) |
Magnifying endoscopy with narrow band imaging to determine the extent of resection in transoral robotic surgery of oropharyngeal cancer | Tateya et al. |
2014 | Japan | Case study | 1 | Left base of tongue lesion identified on ME-NBI. Lesion not identified on CT, MRI or PET. Resection confirmed negative margins | |
Near infra-red light | Intraoperative imaging during minimally invasive transoral robotic surgery using near-infrared light | Scott-Wittenborn et al. | 2018 | USA | Case series | 4 | Diffuse fluorescence of the oropharyngeal tissue achieved within 1 minute with lack of differentiation between healthy and neoplastic tissue. Tumour tissue unable to be identified and study ceased |
HRME | Feasibility of transoral robotic-assisted high-resolution microendoscopic imaging of oropharyngeal squamous cell carcinoma | Patsias et al. |
2015 | USA | Case series | 3 | HRME images obtained during surgery were consistent with histological discrimination between benign and invasive mucosa in all cases reported |
FLIm | Intraoperative delineation of p16+ oropharyngeal carcinoma of unknown primary origin with fluorescence lifetime imaging: Preliminary report | Weyers et al. |
2022 | USA | Case series | 6 | Demonstrated clinical effectiveness in differentiation of benign vs. malignant oropharyngeal tissue with adequate demarcation of margins. Achieved mean specificity and sensitivity of 89% and 96% |
Intraoperative Margin Assessment in Oral and Oropharyngeal Cancer Using Label-Free Fluorescence Lifetime Imaging and Machine Learning | Marsden et al. |
2021 | USA | Cohort study | 53 | ROC AUC of 86% sensitivity and 87% specificity with resection margins identified in 44/53 patients | |
Fluorescence lifetime imaging for intraoperative cancer delineation in transoral robotic surgery | Weyers et al. | 2019 | USA | Case series | 10 | No residual cancer found on post resection scans with statistically significant change seen with P value <0.001 for all 6 FLIm parameters between healthy and cancerous tissue |
OPSCC, oropharyngeal squamous cell carcinomas; NBI, narrow band imaging; CI, confidence interval; US, ultrasound; WLI, white light imaging; ME-NBI, magnifying endoscopy with NBI; CT, computed tomography; MRI, magnetic resonance imaging; PET, positron emission tomography; HRME, high-resolution microendoscope; ROC AUC, receiver operator characteristic area under the curve; FLIm, fluorescence lifetime imaging.
Fifteen studies identified in the search were excluded as they focussed on laryngeal, hypopharyngeal or oral cavity squamous cell carcinoma with a further 6 focussing on mixed subsite disease with nil subgroup analysis. Other studies which focussed on OPSCC patients undergoing TORs for surgical margin outcomes did not focus on the tools of surgical margin delineation and hence were excluded.
The quality of evidence found was low. Of the 9 studies, 6 were observational descriptive studies including 1 case report and 5 case series. Three were cohort studies. No randomized controlled trials (RCTs) were found in the search given the nature of the disease and hence the ethical issues around the conduct of RCT.
Discussion
FSA
Intraoperative surgical margin assessment with FSA has become common practice and is regarded to have a high diagnostic accuracy (11). It is facilitated by the use of cryostat at −30 ℃ which allows the formation of sections from frozen tissue which are at 5–10 µm in range and able to be analysed under the microscope (12). Despite the wide use of this technique, certain factors such as difficulties in tissue processing, inadequate sampling and artefacts can decrease the accuracy of this practice (13). FSA also remains a time-consuming tool in all surgical specialties. Apart from these general pitfalls, FSA in TORS carries its own limitations with monopolar electrocautery being associated with significant tissue disturbance and distortion (14).
Currently, there is limited literature on the use of FSA in TORS procedures and a singular retrospective cohort study was found with 170 participants. Yu et al. [2022] (15) sampled the tumour specimen along the four peripheral borders as well as at the apex of the tumour. There was no specified number of margins required for validation and rather a consensus system between head and neck surgeons was outlined prior to the study. Margins that were seen to be consistent with at least moderate dysplasia, carcinoma in situ or carcinoma were considered positive. It showed that frozen section margin assessment in patients with OPSCC undergoing TORS had an accuracy of 94.1% with a sensitivity of 85.1% and specificity of 97.4% (n=85). Furthermore, the study used “frozen section control biopsies” which were positive intraoperative tumour biopsies to augment this experience. The intention of this strategy was to provide a positive biopsy for comparison of intraoperative margins to allow for more accurate and rapid diagnosis of cellular atypia when compared to standard frozen section control measures. Sensitivity was increased in this cohort of patients with an intraoperative control biopsy (n=85) to 88.9% with a statistically significant difference of 6.1% (95% confidence interval: 3.9–8.3%). There was also a statistically significant difference in reduced time spent in the operating theatre. Out of the total cohort of 170 patients, only 11 required additional margin sampling. Although this is the only study which has investigated FSA in TORS patients with OPSCC, the high diagnostic accuracy with or without the control biopsies concurs with all previous studies in other modalities of surgery (16,17) and hence is an established approach for margin sampling intraoperatively. No further studies were found or excluded that reported on specimen based frozen sections rather than at risk margins.
IUS
IUS remains a novel technique in TORS procedures but has been well established in reducing rates of positive surgical margins in urological procedures (18). It allows for assessment to happen in real time, in vivo prior to tissue excision. In TORS, it can be utilised with different probes depending on access site. The robotic arms are removed whilst leaving the camera in position and the ultrasound probes are inserted alongside the camera with the images being displayed directly on the robotic console. This arrangement allows for the potential of up to two robotic arms inside concurrently with the ultrasound probe, dependent on the robotic console. A singular case series by Clayburgh et al. [2016] (19) demonstrated that the ultrasound augmented surgical experience by being able to visualise surgical margins between tumours and healthy tissue as an echogenic line. In four patients with OPSCC, ultrasound was also able to visualise deep margins of the tumour to an accuracy of 1–2 mm, allowing the surgeons to perform a wide resection of clear margins. In this study, cases included base of tongue (BOT), tonsillar and oropharyngeal carcinomas of which, all returned negative surgical margins. Given the tendency to perform a compartmental resection into the parapharyngeal space for tonsillar tumours, the use potential is larger in BOT cancers. Although, this is a novel technique in the assessment of surgical margins, it is one of the only techniques with the advantage of identifying deep surgical margins as well as the ability to identify vasculature. This significantly decreases the risks of haemorrhage in tongue base resection with close proximity to the dorsal lingual vessels.
Multiple studies and reports look at the future of IUS with direct integration into the robotic console. Unger et al. [2021] (20) developed a system utilising two different robotic arms where one can be used in positioning a mobile ultrasound probe to plan the intervention and the second arm allows for the therapeutic intervention to be carried out. Furthermore, Harbin Institute of Technology have recently proposed an ultrasound guided percutaneous prostate robotic system with eight joints (21). This proposed model allows for independent control of ultrasound probe manipulation and needle positioning using one of the arms. Both models remain in the research and development phase with a long way to go from prototype to an evidence-based medical device. Further research is required for the integration of these devices in robotic consoles used for head and neck surgery.
Narrowband imaging
NBI already has been a validated tool in the early detection of oropharyngeal, hypopharyngeal and laryngeal tumours (22-24). The technology uses a narrow band spectrum filter to reduce illumination to two specific wavelengths of light: blue (415 nm) and green (540 nm). These have the highest absorption spectrum for haemoglobin and hence enhance the mucosal and submucosal microvascular patterns to be able to create the highest contrast picture when compared to white light imaging (WLI) which includes all wavelengths of light. This allows for the detection of abnormal vasculature and hints at tumour margins.
Only two studies were found that evaluated the use of this technology in TORS including a prospective cohort study and a case report. Vicini et al. [2015] (25) showed a statistically significant higher rate of negative lateral margins at 87.9% when using NBI when compared to WLI at 57.9%. This included a cohort of 58 patients undergoing TORS with 44 having biopsy proven OPSCC. The sensitivity and specificity of NBI was 72.5% and 66.7% respectively. Furthermore, Tateya et al. [2014] (26) showed a case report of a 55-year-old male with OPSCC with a 1.5-cm lesion at left BOT. NBI clearly recognised the area as brown mucosal pattern with scattered brown dots representing abnormal vasculature which was not identified by WLI, computed tomography (CT), magnetic resonance imaging (MRI) or positron emission tomography (PET) imaging modalities. Both study designs confirmed that NBI improves visualisation of lateral superficial margins of resection. A weakness of this imaging modality remains the inability to capture and assist with deep margin resections. Anecdotally, surgeons have also expressed issues with intraoperative use of this technique due to its absorption by haemoglobin. This requires a blood-free mucosal surface which is inherently obscured once resection commences. Given the paucity of evidence, further research is required to validate this imaging modality for superficial margins of resection and ways of reducing the issues with view secondary to mucosal bleeding.
FLIm
FLIm focusses on the emission of red light by a molecule after the absorption of the same light at a shorter wavelength. The characteristics of the tissue, molecular structures and metabolism leads to differences in the emission wavelength which helps to create a stark optical contrast between different tissues (27). This allows for the identification of the demarcation between healthy and neoplastic tissue given their altered metabolism state and is termed autofluorescence. Similar technique can be conducted using fluorescent tagged probes which rely on intravenous injection and accumulation in tumour tissue secondary to leaky capillaries. A range of fluorescent tagged probes are available and further probes remain in development which show promise. The intraoperative and real time dynamic of FLIm allows it to circumvent the delayed process of FSA. This technique is still in experimental stages given the significant challenges associated with equipment required to perform the technique. Three studies including two case series and one cohort study was found evaluating this technique in OPSCC patients undergoing TORS procedures.
Weyers et al. [2022] (28) demonstrated clinical effectiveness in differentiation of benign vs malignant oropharyngeal tissue with a sensitivity and specificity of 96% and 89% respectively. This was backed by a previous study (29) conducted by the same author in 2019 with statistically significant P value <0.001 for six FLIm parameters in differentiating cancerous and benign tissue. Marsden et al. [2021] (30) successfully identified resection margins in 44 out of the 53 patients with a calculated receiver operator characteristic area under the curve of 86% sensitivity and 87% specificity. The FLIm technique was accurate to the depth of 250 µm or less given its limitations (28). Given the promising results in these limited studies, once the FLIm technique is validated extensively, it has potential in heavily assisting with cancer delineation.
NIR
NIR involves the injection of indocyanine green dye which has a high affinity for blood borne proteins and hence, fluoresces vasculature when illuminated by NIR. Given known tumour characteristics of abnormal vasculature, it serves to highlight and improve visualisation of such neoplastic tissue with a qualitative difference. It has demonstrated effective utility in renal and adrenal tumour resections (31,32). A single case series was found evaluating this technique in TORS patients with OPSCC. Scott-Wittenborn et al. [2017] (33) demonstrated no effect in differentiating between benign and neoplastic tissue in the oropharynx. Due to these unpromising results, the study was ceased after the 6th patient. The particularly high vascularity of the oropharyngeal mucosa and lymphoid tissue could partly explain the above findings, given this does not allow for a clinically significant differentiation in fluorescence. Additionally, the rapid clearance of these intravenously administered agents further impairs the desired effects of this imaging modality. Further research in a larger case series is required to look at the viability of this technique.
High-resolution microendoscope (HRME)
HRME employs the use of topical fluorescent nuclear contrast for visualisation of epithelial structures at a subcellular level of resolution with a flexible fibre optic probe. It has been previously validated for detection of head and neck squamous cell carcinoma ex vivo with sensitivity and specificity of 98% and 92% (34,35). In a single case series conducted by Patsias et al. [2015] (36), HRME images showed features that were consistent with histological assessment and could discriminate between benign mucosa and invasive cancer. It contrasted nuclei which was consistently spaced and regular sized with enlarged, crowded nuclei distorting the cellular architecture. It was limited by a depth of penetration to 50 µm, making it challenging to identify submucosal spread. A limitation of HRME remains the need for tissue contact with the flexible fibre optic probe which can prove challenging in submucosal locations, tissue necrosis and hyperkeratosis. No further studies were found which investigated OPSCC in TORS patients.
Conclusions
Image guided adjuncts to TORS including IUS, NBI, FLIm, NIR and HRME are all novel and promising adjuncts in use of margin delineation intraoperatively. They all serve to circumvent the intraoperative delays of frozen section sampling by providing real-time data. All techniques establish superficial margins in real time assessment but still lack the ability for deep margin assessment which the FSA has been validated for previously. Further research with larger cohort studies is required to validate each tool given the low quality of evidence found in this study. Furthermore, studies combining these strategies for superficial margin delineation and deep margin assessment may also be helpful. Other strategies previously investigated in head and neck cancer open surgery including but not limited to elastic scattering spectroscopy, Raman spectroscopy and optical coherence tomography require further engineering and validation with TORS procedures.
Acknowledgments
Our abstract has been presented at the Royal Australian College of Surgeons Annual Congress in 2023 in Adelaide, South Australia.
Funding: None.
Footnote
Reporting Checklist: The authors have completed the PRISMA-ScR reporting checklist. Available at https://www.theajo.com/article/view/10.21037/10.21037/ajo-24-9/rc
Peer Review File: Available at https://www.theajo.com/article/view/10.21037/ajo-24-9/prf
Conflicts of Interest: All authors have completed the ICMJE uniform disclosure form (available at https://www.theajo.com/article/view/10.21037/ajo-24-9/coif). The authors have no conflicts of interest to declare.
Ethical Statement: The authors are accountable for all aspects of the work in ensuring that questions related to the accuracy or integrity of any part of the work are appropriately investigated and resolved. Ethical approval was not required given the nature of the scoping review.
Open Access Statement: This is an Open Access article distributed in accordance with the Creative Commons Attribution-NonCommercial-NoDerivs 4.0 International License (CC BY-NC-ND 4.0), which permits the non-commercial replication and distribution of the article with the strict proviso that no changes or edits are made and the original work is properly cited (including links to both the formal publication through the relevant DOI and the license). See: https://creativecommons.org/licenses/by-nc-nd/4.0/.
References
- Chimenos-Küstner E, Marques-Soares MS, Schemel-Suárez M. Aetiopathology and prevention of oropharyngeal cancer. Semergen 2019;45:497-503. [PubMed]
- Lorenzoni V, Chaturvedi AK, Vignat J, et al. The Current Burden of Oropharyngeal Cancer: A Global Assessment Based on GLOBOCAN 2020. Cancer Epidemiol Biomarkers Prev 2022;31:2054-62. [Crossref] [PubMed]
- Castellano A, Sharma A. Systematic Review of Validated Quality of Life and Swallow Outcomes after Transoral Robotic Surgery. Otolaryngol Head Neck Surg 2019;161:561-7. [Crossref] [PubMed]
- Gorphe P, Simon C. A systematic review and meta-analysis of margins in transoral surgery for oropharyngeal carcinoma. Oral Oncol 2019;98:69-77. [Crossref] [PubMed]
- Woolgar JA, Triantafyllou A. A histopathological appraisal of surgical margins in oral and oropharyngeal cancer resection specimens. Oral Oncol 2005;41:1034-43. [Crossref] [PubMed]
- Bernier J, Cooper JS, Pajak TF, et al. Defining risk levels in locally advanced head and neck cancers: a comparative analysis of concurrent postoperative radiation plus chemotherapy trials of the EORTC (#22931) and RTOG (# 9501). Head Neck 2005;27:843-50. [Crossref] [PubMed]
- White H, Ford S, Bush B, et al. Salvage surgery for recurrent cancers of the oropharynx: comparing TORS with standard open surgical approaches. JAMA Otolaryngol Head Neck Surg 2013;139:773-8. [Crossref] [PubMed]
- Funk RK, Moore EJ, García JJ, et al. Risk factors for locoregional relapse after transoral robotic surgery for human papillomavirus-related oropharyngeal squamous cell carcinoma. Head Neck 2016;38:E1674-9. [Crossref] [PubMed]
- Peters MD, Godfrey CM, Khalil H, et al. Guidance for conducting systematic scoping reviews. Int J Evid Based Healthc 2015;13:141-6. [Crossref] [PubMed]
- Tricco AC, Lillie E, Zarin W, et al. PRISMA Extension for Scoping Reviews (PRISMA-ScR): Checklist and Explanation. Ann Intern Med 2018;169:467-73. [Crossref] [PubMed]
- Baddour HM Jr, Magliocca KR, Chen AY. The importance of margins in head and neck cancer. J Surg Oncol 2016;113:248-55. [Crossref] [PubMed]
- Jaafar H. Intra-operative frozen section consultation: concepts, applications and limitations. Malays J Med Sci 2006;13:4-12. [PubMed]
- Smithers FAE, Haymerle G, Palme CE, et al. A prospective study of intraoperative assessment of mucosal squamous cell carcinoma margins in the head and neck. Head Neck 2021;43:590-600. [Crossref] [PubMed]
- Kakarala K, Faquin WC, Deschler DG. A comparison of histopathologic margin assessment after steel scalpel, monopolar electrosurgery, and ultrasonic scalpel glossectomy in a rat model. Laryngoscope 2010;120:S155. [Crossref] [PubMed]
- Yu AC, Afework DD, Goldstein JD, et al. Association of Intraoperative Frozen Section Controls With Improved Margin Assessment During Transoral Robotic Surgery for Human Papillomavirus-Positive Oropharyngeal Squamous Cell Carcinoma. JAMA Otolaryngol Head Neck Surg 2022;148:1029-37. [Crossref] [PubMed]
- Nayanar SK. Frozen Section Evaluation in Head and Neck Oncosurgery: An Initial Experience in a Tertiary Cancer Center. Turk Patoloji Derg 2019;35:46-51. [PubMed]
- Thomas Robbins K, Triantafyllou A, Suárez C, et al. Surgical margins in head and neck cancer: Intra- and postoperative considerations. Auris Nasus Larynx 2019;46:10-7. [Crossref] [PubMed]
- Ukimura O, Magi-Galluzzi C, Gill IS. Real-time transrectal ultrasound guidance during laparoscopic radical prostatectomy: impact on surgical margins. J Urol 2006;175:1304-10. [Crossref] [PubMed]
- Clayburgh DR, Byrd JK, Bonfili J, et al. Intraoperative Ultrasonography During Transoral Robotic Surgery. Ann Otol Rhinol Laryngol 2016;125:37-42. [Crossref] [PubMed]
- Unger M, Berger J, Melzer A. Robot-Assisted Image-Guided Interventions. Front Robot AI 2021;8:664622. [Crossref] [PubMed]
- Yan J, Pan B, Fu Y. Ultrasound-guided prostate percutaneous intervention robot system and calibration by informative particle swarm optimization. Front Mech Eng 2022;17:3. [Crossref]
- Ansari UH, Wong E, Smith M, et al. Validity of narrow band imaging in the detection of oral and oropharyngeal malignant lesions: A systematic review and meta-analysis. Head Neck 2019;41:2430-40. [Crossref] [PubMed]
- Hu L, Miao Z, Sun C. Diagnostic performance of NBI in post-treatment follow up for laryngeal cancer: A systematic review and meta-analysis. Am J Otolaryngol 2022;43:103530. [Crossref] [PubMed]
- Kim DH, Kim SW, Lee J, et al. Narrow-band imaging for screening of oral premalignant or cancerous lesions: A systematic review and meta-analysis. Clin Otolaryngol 2021;46:501-7. [Crossref] [PubMed]
- Vicini C, Montevecchi F, D'Agostino G, et al. A novel approach emphasising intra-operative superficial margin enhancement of head-neck tumours with narrow-band imaging in transoral robotic surgery. Acta Otorhinolaryngol Ital 2015;35:157-61. [PubMed]
- Tateya I, Ishikawa S, Morita S, et al. Magnifying endoscopy with narrow band imaging to determine the extent of resection in transoral robotic surgery of oropharyngeal cancer. Case Rep Otolaryngol 2014;2014:604737. [Crossref] [PubMed]
- Datta R, Heaster TM, Sharick JT, et al. Fluorescence lifetime imaging microscopy: fundamentals and advances in instrumentation, analysis, and applications. J Biomed Opt 2020;25:1-43. [Crossref] [PubMed]
- Weyers BW, Birkeland AC, Marsden MA, et al. Intraoperative delineation of p16+ oropharyngeal carcinoma of unknown primary origin with fluorescence lifetime imaging: Preliminary report. Head Neck 2022;44:1765-76. [Crossref] [PubMed]
- Weyers BW, Marsden M, Sun T, et al. Fluorescence lifetime imaging for intraoperative cancer delineation in transoral robotic surgery. Transl Biophotonics 2019;1:e201900017. [Crossref] [PubMed]
- Marsden M, Weyers BW, Bec J, et al. Intraoperative Margin Assessment in Oral and Oropharyngeal Cancer Using Label-Free Fluorescence Lifetime Imaging and Machine Learning. IEEE Trans Biomed Eng 2021;68:857-68. [Crossref] [PubMed]
- Sound S, Okoh AK, Bucak E, et al. Intraoperative tumor localization and tissue distinction during robotic adrenalectomy using indocyanine green fluorescence imaging: a feasibility study. Surg Endosc 2016;30:657-62. [Crossref] [PubMed]
- Tobis S, Knopf J, Silvers C, et al. Near infrared fluorescence imaging with robotic assisted laparoscopic partial nephrectomy: initial clinical experience for renal cortical tumors. J Urol 2011;186:47-52. [Crossref] [PubMed]
- Scott-Wittenborn N, Jackson RS. Intraoperative imaging during minimally invasive transoral robotic surgery using near-infrared light. Am J Otolaryngol 2018;39:220-2. [Crossref] [PubMed]
- Muldoon TJ, Anandasabapathy S, Maru D, et al. High-resolution imaging in Barrett's esophagus: a novel, low-cost endoscopic microscope. Gastrointest Endosc 2008;68:737-44. [Crossref] [PubMed]
- Pierce MC, Vila PM, Polydorides AD, et al. Low-cost endomicroscopy in the esophagus and colon. Am J Gastroenterol 2011;106:1722-4. [Crossref] [PubMed]
- Patsias A, Giraldez-Rodriguez L, Polydorides AD, et al. Feasibility of transoral robotic-assisted high-resolution microendoscopic imaging of oropharyngeal squamous cell carcinoma. Head Neck 2015;37:E99-102. [Crossref] [PubMed]
Cite this article as: Goel S, Krishnan G, Krishnan S, Hodge JC, Foreman A. Transoral robotic surgery for oropharyngeal squamous cell carcinoma: a scoping review of novel techniques in margin assessment. Aust J Otolaryngol 2024;7:25.