An audit of outcomes of cochlear implantation in patients following temporal bone fracture
Introduction
Temporal bone fractures are a common occurrence in trauma, present in 3% of all trauma patient (1). High-energy blunt head trauma causes the majority of temporal bone fractures, with half of fractures due to motor vehicle crashes, a third due to falls, and ~10% due to assault (2).
Temporal bone fractures were classically described as either longitudinal (80%) or transverse (20%) (3), however this classification failed to correlate well with clinical sequelae. The preferred contemporary classification system nowadays is to instead classify a fracture as either otic capsule sparing or otic capsule violating, which more accurately predicts the outcomes of temporal bone trauma (2). Up to 10% of patients may sustain bilateral fractures (4).
Sensorineural hearing loss occurs in 14–23% of temporal bone fractures, with 20–55% having mixed hearing loss (5). Otic violating fractures have a rate of sensorineural hearing loss 7 to 25 times greater than otic sparing fractures (2,6). Interruption of the otic capsule often causes a complete sensorineural hearing loss, occurring in 14% of all temporal bone fractures (5). In the setting of a unilateral fracture, the resulting single sided deafness affects sound localisation, speech comprehension in noisy environments, spatial awareness and can have significant quality of life, vocational and social impacts. Those patients unfortunate enough to have bilateral otic capsule violating fractures with hearing loss clearly suffer a profound quality of life imposition.
Temporal bone fractures may cause severe loss of hair cells, ganglion cells and other supporting cells in the inner ear (7). Head trauma may still cause sensorineural hearing loss even in the absence of a temporal bone fracture, with similar histopathological findings of hair cell loss, atrophy of the stria vascularis, cochlear hydrops and reduced spiral ganglion cell counts following trauma (8).
Delayed sensorineural loss may also occur in the form of sympathetic hearing loss, affecting up to 10% of patients (9). This represents an autoimmune reaction, due to exposure of inner ear antigens following trauma, and may result in hearing loss in months to years after trauma.
In patients with severe to profound or complete sensorineural hearing loss, cochlear implantation represents an opportunity for hearing rehabilitation. However, implantation in this group poses challenges, given the fracture of the temporal bone may distort the normal anatomy, and cause intracochlear fibrosis and osteoneogenesis, making implantation difficult. The aim of this study is to evaluate cochlear implantation in this group, to determine whether audiological outcomes and adverse events are affected by the presence of a previous temporal bone fracture. We also examined the effect of factors including fracture type, evidence of ossification on pre-operative imaging, age at implantation, and timing to implantation on these outcomes. We present this article in accordance with the STROBE reporting checklist (available at https://www.theajo.com/article/view/10.21037/ajo-23-12/rc).
Methods
The study was conducted in accordance with the Declaration of Helsinki (as revised in 2013). This retrospective study was approved by the Human Research Ethics Committee (No. 18/1367HS). Because of the retrospective nature of the research, the requirement for informed consent was waived. The Cochlear Implant clinic at the Royal Victorian Eye and Ear Hospital serves all Victorian patients.
The Cochlear Implant database was analysed for implantation in patients with an indication reported as head injury or trauma which yielded 48 patients. A chart review was performed, to determine clear evidence of temporal bone fractures on pre-operative imaging. A total of 14 adult patients with post lingual hearing loss were identified to have had cochlear implantation following temporal bone fracture between January 2000 to June 2020, with audiological data available for analysis for 13 patients.
A control group was also identified from the database, with 1,414 adult patients with post lingual hearing loss implanted from January 2000 to June 2020 with otherwise normal cochlear anatomy. Patients were excluded from the study if they had documented otosclerosis as aetiology, were non-English speakers, patients with psychiatric or medical conditions precluding formal testing, and those patients that received a research device.
All patients were discussed in the Cochlear Implant Multidisciplinary Meeting prior to implantation. Computed tomography (CT) petrous temporal bone and magnetic resonance imaging (MRI) scans were performed in all patients for workup and operative planning. Follow up was performed at 3 and 12 months to assess audiological outcomes and ongoing use.
Patients underwent speech perception assessment before and after the procedure. Testing included open-set monosyllabic word testing in quiet [consonant-vowel-consonant (CVC) words] scored on the basis of phonemes and words correct. Pure tone audiograms, CVC word and phoneme scores were recorded pre-operatively. Post operative assessment included CVC word and phoneme scores, and was recorded at 3 and 12 months post implantation. Speech perception testing was performed at 65 dB. Hearing status in the contralateral ear, pre-implantation duration of deafness, and ongoing use at last review were also recorded.
Patient charts were reviewed to identify the type of device implanted, as well as any intraoperative and post operative adverse events.
Successful implantation was defined as a CVC phoneme score of 55% or greater at 65 dB, with ongoing utilisation of the implant. In 2016 our centre published data from a large cohort of patients (n=382 patients), and found that 75% (n=288 patients) of adults met this definition of success after 12 months of use (10).
An intermediate outcome was recorded in those patients with an improvement in phoneme score of greater than 10%, but overall score less than 55%, with ongoing use. An unsuccessful outcome was defined as no ongoing use or improvement in phoneme score of less than 10% from baseline.
Student’s two sample t-test was used to compare audiological outcomes between groups, and Pearson pairwise correlation was used to test for correlation between time to implantation or age at implantation and audiological outcomes. For pairwise comparisons, r value of >0.5 was considered strong, 0.3–0.5 moderate and 0.1–0.3 weak correlation. Statistical analysis was performed using XLStat software.
Results
A total of 14 patients were identified having undergone implantation in the setting of a previous temporal bone fracture, with subsequent post lingual hearing loss. The results are summarised in Table 1. Patient age at insertion ranged from 21 to 80 years old. Unilateral temporal bone fractures were present in nine patients, due to fall (3 patients), motor vehicle accident (2 patients), train accident (2 patients), bicycle accident (1 patient) and unknown cause (1 patient). Five patients had bilateral fractures, due to assault (2 patients), fall (1 patient) and motor vehicle accident (2 patients). Three of five (60%) patients with bilateral fractures underwent bilateral implantation.
Table 1
Patient | Age at implantation (years) | Hearing loss | Fracture location | Imaging features | Implantation | Side | Time to implantation (years) | Adverse event | CVC word scores | CVC phoneme scores | |||
---|---|---|---|---|---|---|---|---|---|---|---|---|---|
3 months (%) | 12 months (%) | 3 months (%) | 12 months (%) | ||||||||||
1 | 64 | Bilateral | Sparing | Nil significant | Unilateral | Left | 20 | None | 28 | 34 | 55 | 60 | |
2 | 41 | Bilateral | Sparing | Nil significant | Unilateral | Left | 15 | None | 40 | LTFU | 70 | LTFU | |
3 | 63 | Bilateral | Violating | Fracture line involving basal turn | Unilateral | Right | 16 | Partial insertion | 14 | 8 | 39 | 29 | |
4 | 36 | Bilateral | Bilateral: right, violating; left, sparing | Right: fracture line involving basal turn | Bilateral | Right | 0.5 | Failed initial insertion. Basal turn drill out required | Not recorded† | ||||
Left | None | 64 | 60 | 80 | 82 | ||||||||
5 | 67 | Bilateral | Violating | Scala tympani fibrosis | Unilateral | Right | 35 | Scala vestibuli insertion | 0 | Non-user | 11 | Non-user | |
6 | 47 | Bilateral | Bilateral: right, violating; left, sparing | Left: calcification of basal turn involving scala tympani on CT | Unilateral; right | Right | 26 | None | 26 | 52 | 51 | 77 | |
7 | 80 | Bilateral | Violating | Nil significant | Unilateral | Right | 1.5 | None | 0 | Non-user | 4 | Non-user | |
8 | 56 | Bilateral | Bilateral: right, violating; left, violating | Nil significant | Bilateral | Left | 0.5 | None | 12 | 38 | 8 | 39 | |
Right | None | 14 | 16 | 40 | 43 | ||||||||
9 | 40 | Bilateral | Bilateral: right, sparing; left, sparing | Nil significant | Unilateral | Right | 18 | None | 70 | 88 | 83 | 95 | |
10 | 23 | Single sided deafness | Sparing | Nil significant | Unilateral | Right | 1 | None | 56 | 65 | 77 | 84 | |
11 | 74 | Bilateral | Violating | Nil significant | Unilateral | Right | 18 | None | 40 | 42 | 66 | 69 | |
12 | 71 | Bilateral | Violating | Nil significant | Unilateral | Right | 50 | Facial nerve stimulation | 0 | 0 | 0 | 0 | |
13 | 21 | Bilateral | Bilateral: left, violating; right, sparing | Left: fracture line involving cochlear | Bilateral | Left | 1 | Partial insertion | 50 | LTFU | 76 | LTFU | |
Right | None | 68 | 84 | ||||||||||
14 | 23 | Single sided deafness | Violating | Fracture line involving basal turn, scala tympani fibrosis | Unilateral | Right | 8 | Scala vestibuli insertion. Facial nerve stimulation | Non-user | Non-user | Non-user | Non-user |
†, independent hearing outcomes were not scored for the right ear in patient 4, rather they were assessed with the left implant and bilateral implants. CVC, consonant-vowel-consonant; LTFU, lost to follow up; CT, computed tomography.
Of a total of 19 temporal bone fractures, eight were otic capsule sparing, while eleven were otic capsule violating. There was evidence of cochlear involvement of the fracture line in eight of the otic capsule violating fractures. A total of 17 implantations were performed.
The duration of pre-implantation deafness varied greatly from 6 months to 50 years, with a median of 16 years. Two patients were implanted for single side deafness, the remainder had bilateral hearing loss.
Pre-curved Contour advance electrodes were preferred for the majority of insertions [Cochlear CI 24 RE (CA) or CI 512 electrodes] implanted in 13 patients (16 insertions). The remaining patient received a Cochlear CI 522 straight slim electrode.
Adverse outcomes only occurred in the presence of an otic capsule violating fracture. One patient with bilateral fractures (patient 4) had an initial failed unilateral implantation, due to false insertion into the hypotympanic cell tract in the presence of ossification of the basal turn. This patient was successfully reimplanted ipsilaterally, requiring a drill out of the basal turn and proceeded to subsequent contralateral implantation. Two patients (patients 5 and 14) required scala vestibuli insertion due to ossification of the scala tympani. Pre-operative MRI demonstrated fibrosis of the scala tympani in both patients that underwent scala vestibuli insertion. A further two patients (patients 3 and 13) only had partial insertion, also due to ossification which was evident on pre-operative imaging.
Two patients experienced facial nerve stimulation initially. With implant programming this was able to be alleviated in both patients. Interestingly, in one patient this occurred in the tip electrodes, while in the other patient it was the basal electrodes causing facial nerve stimulation. Both patients were implanted with CI 512, with a perimodiolar electrode array which is known to be protective against facial nerve stimulation (11). In one patient (patient 14), there was fibrosis of the basal turn on preoperative MRI which required scala vestibuli insertion, while the other patient (patient 12) had standard insertion with a fluid filled cochlear on preoperative MRI.
Audiological outcomes were available for 13 of 14 patients, with one patient lost to follow up immediately, with no ongoing use of their implant. Four patients only had 3-month data available, with two patients lost to follow up and two patients no longer using their implant at 12 months.
The control group mean CVC word scores were 36% at 3 months and 44% at 12 months. The control group mean CVC phoneme scores were 58% at 3 months and 65% at 12 months. The mean post implantation CVC word score for patients with temporal bone fractures was 34% (range, 0–88%), while the mean CVC phoneme score was 53.54% (range, 0–95%).
CVC word and phoneme scores at last follow up were compared to the control group mean (see Table 2). There was no statistical difference in the phoneme scores between implantation in otic capsule sparing fractures compared to the control group (mean 78.8% vs. 63.7%, P=0.097). Otic capsule violating fractures had worse phoneme scores than both the control group (33.8% vs. 63.7%, P=0.0005), and the otic capsule sparing group (33.8% vs. 78.8%, P=0.002).
Table 2
Audiological outcome | Otic capsule sparing fracture, % | Otic capsule violating fracture, % | Control group, % | P1 | P2 | P3 |
---|---|---|---|---|---|---|
Mean CVC word score at last follow up | 58.1 | 15.5 | 41.9 | 0.091 | 0.003* | 0.001* |
Mean CVC phoneme score at last follow up | 78.8 | 33.8 | 63.7 | 0.097 | 0.0005* | 0.002* |
P1: otic capsule sparing fractures compared to control group; P2: otic capsule violating fractures compared to control group; P3: otic capsule sparing fractures compared to otic violating sparing fractures. *, P values reach statistical significance. CVC, consonant-vowel-consonant.
There was a statistically significant strong correlation between age at implantation and both CVC word (r=−0.763; P=0.001) and phoneme scores (r=−0.773; P=0.001) on Pearson correlation, with audiological scores reducing with advancing age (see Figure 1). While there appeared to be a similar trend of reducing audiological scores with increased time to implantation, this did not reach statistical significance [CVC word (r=−0.314; P=0.254), CVC phoneme scores (r=−0.422; P=0.117)]. A number of patients with delayed implantation performed poorly on both CVC word and phoneme scores (see Figure 2). Notably however, there was still benefit seen for patients implanted up to 26 years post onset of hearing loss.
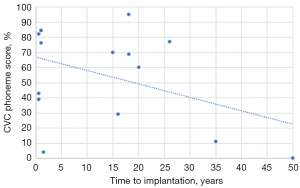
Successful implantation (phoneme ≥55% with ongoing use) was recorded in 8 of 14 patients (57%). The rate of successful implantation was 100% for otic capsule sparing fractures. Implantation in otic capsule violating fractures was successful in 30%, had an intermediate outcome in 20% and was unsuccessful in 50%. All patients with an unsuccessful outcome had an otic violating fracture in the implanted ear, with four having bilateral hearing loss. Four patients ceased implant use, while one still found benefit from their implant, despite reduced speech perception scores.
Of the four patients which ceased implant use, in one patient (patient 12) this was likely due to the prolonged duration of deafness, with his temporal bone fracture having occurred 50 years earlier. This patient was implanted in the setting of new moderate-profound contralateral sensorineural hearing loss secondary to sudden sensorineural hearing loss. At 12 months their CVC word and phoneme scores in the implanted ear were 0% and 0% respectively. They have since returned to using a Bi-CROS hearing aid with reasonable satisfaction. The second patient (patient 5) underwent scala vestibuli insertion, experienced facial nerve stimulation, and unfortunately also had a poor audiological outcome, with 0% word scores and 11% phoneme scores at the 3-month follow up. They had a 35-year interval between fracture and implantation, and as such neural atrophy may be the primary cause of the poor outcome rather than the scala vestibuli insertion itself. They were implanted in the setting of progressive presbyacusis in the contralateral ear. Despite consistent use for 9 months, they were unable to adjust to the new sound quality, did not receive any perceptible benefit and discontinued use. The third patient that ceased implant use (patient 7) also had a poor audiological outcome, with CVC word and phoneme scores of 0% and 4% respectively, and they did not persist with use in the setting of advancing dementia. The fourth patient failed to attend any follow up appointments after moving interstate, with no post-op audiological assessment performed.
Discussion
The data from our centre demonstrates a significant difference in the outcomes between otic capsule sparing and otic capsule violating fractures. Our study demonstrates a higher rate of success when the fracture does not violate the otic capsule, which is consistent with a systematic review by Cowan et al. (12). Audiological outcomes in the setting of an otic capsule violating fracture are significantly worse than the general implant population. Implants inserted into a fractured otic capsule were still utilized in 50% of cases, suggesting that as these patients have profound or total hearing loss, even suboptimal audiological outcomes may provide significant quality of life benefits in the setting of bilateral hearing loss.
There was a statistically significant negative correlation between age at implantation and audiological outcomes, in the absence of a significant correlation between time to implantation and outcomes. Hearing assessment may often be delayed in these patients, as ~90% have concurrent intracranial injuries, 9% have cervical spine injuries, and are often associated with other severe injuries (13). While delays to implantation may limit efficacy in the setting of neural atrophy, a number of patients with prolonged time to implantation still had significant benefit. Advancing age may reduce audiological outcomes, but hearing rehabilitation is still valuable in these patients for protecting neurocognitive functioning (14).
All insertions which were complicated by facial nerve stimulation, failed or partial insertion, or required scala vestibuli insertion, occurred in otic capsule violating fractures. Imaging was predictive in all cases of insertion difficulty, with evidence of cochlear involvement by fracture or ossification evident.
CT imaging may overlook ossification. Seidman et al. (15) found intraoperative ossification that was not identified on high-resolution computed tomography (HRCT) in 22% of cases. In 5 of 18 cases in their experience, the round window membrane was found to be ossified with a patent cochlear lumen. In 7 of 18 cases, ossification was found to extend for up to 2 mm into the cochlear lumen. In 6 cases, 3–9 mm of the basal turn was occluded. In all of these cases, the cochlear lumen appeared patent on HRCT. As such, pre-operative imaging with both CT and MRI is essential in these patients, as it can predict the occurrence of perioperative adverse events by identifying both bony obstruction and fluid signal abnormalities.
Facial nerve stimulation is reported to occur in 1–14.9% of cochlear implantation across all indications (16). In our group, the rate was 11.7% (2 insertions). Espahbodi et al. (17) suggested that fracture lines may provide a lower impedance pathway to the adjacent facial nerve and reduce the threshold for facial nerve stimulation. Fortunately for our patient group, facial nerve stimulation was able to be overcome with programming changes and did not impair ongoing use. For one patient, facial nerve stimulation occurred despite scala vestibuli insertion. Bigelow et al. (18), in a study of temporal bone dissection, demonstrated that the scala vestibuli was more distant from the labyrinthine segment of the facial nerve than the scala tympani. Despite this, scala vestibuli insertion does not absolutely protect from facial nerve insertion. A systematic review by Eastwood et al. (19), found that 50% of patients with facial nerve stimulation following implantation in the setting of temporal bone fracture did require reimplantation. Facial nerve stimulation has been shown also to be a problem in patients undergoing implantation for advanced otosclerosis, which is another group where aberrant electrical conduction may take place (20). In the presence of otosclerosis, perimodiolar electrodes have been shown to greatly reduce the incidence of facial nerve stimulation (21,22). The incidence of facial nerve stimulation in this group should not be a barrier to implantation, however potential recipients should have informed consent regarding the elevated risk and potential need for reimplantation. Perimodiolar implants should be preferred in the setting of previous temporal bone fracture.
Scala vestibuli insertion was necessitated in two patients due to the previous fracture. A previous study from our centre demonstrated a 10.5% decrease in phoneme scores for scala vestibuli insertion (23), with a mean 12-month phoneme score for planned scala vestibuli recipients of 46.1%. Trudel et al. (24) have demonstrated equivalent audiological outcomes for scala vestibuli insertion when compared to scala tympani insertion in the presence of ossification. In the presence of ossification of the scala tympani, scala vestibuli insertion is a reasonable alternative still providing valuable hearing rehabilitation.
While not observed in our group, it is also important to consider the risk of perioperative meningitis, which may be increased in the setting of a fracture. There were 2 cases of meningitis across 96 implants in the review by Eastwood et al. (19), which is significantly higher than the overall rate of post operative meningitis (0.25%) (25).
It is also important to emphasise that not all patients are suitable for cochlear implantation. A thorough pre operative work up, assessment of the patients medical and social status, multidisciplinary imaging review and appropriate pre-counselling regarding the goals and expectations of the patient should be performed for all potential recipients. There is a proportion of individuals with temporal bone fractures that may be ineligible for implantation due to one or more factors.
Implantation in the setting of single sided deafness should be considered cautiously, and may be contraindicated in the presence of an otic capsule violating fracture given the poorer audiological outcomes. Only two patients with single sided deafness were implanted in our study, with one patient with an otic capsule sparing fracture performing well (CVC word score 65% and CVC phoneme score 84% at 12 months), while one patient with an otic capsule violating fracture failed to attend any follow up.
Conclusions
Cochlear implantation following temporal bone fracture is safe, and while technical difficulties may be encountered in the presence of an otic capsule violating fracture, implantation may provide significant hearing and communication benefits, even when occurring several years post injury. In patients with fractures violating the otic capsule, audiological outcomes are worse. Pre-operative imaging is essential to provide a recommendation regarding implantation, and to allow for surgical planning. Where there is evidence of fibrous or bony obstruction on imaging, this indicates a potentially difficult insertion and poorer prognosis, and as such careful assessment needs to be made to determine if implantation is still appropriate.
Acknowledgments
Funding: None.
Footnote
Reporting Checklist: The authors have completed the STROBE reporting checklist. Available at https://www.theajo.amegroups.com/article/view/10.21037/ajo-23-12/rc
Data Sharing Statement: Available at https://www.theajo.com/article/view/10.21037/ajo-23-12/dss
Peer Review File: Available at https://www.theajo.com/article/view/10.21037/ajo-23-12/prf
Conflicts of Interest: All authors have completed the ICMJE uniform disclosure forms (available at https://www.theajo.com/article/view/10.21037/ajo-23-12/coif). JMG receives consultancy fees for workshops, R&D, expert opinions from Cochlear Ltd. The other authors have no conflicts of interest to declare.
Ethical Statement: The authors are accountable for all aspects of the work in ensuring that questions related to the accuracy or integrity of any part of the work are appropriately investigated and resolved. The study was conducted in accordance with the Declaration of Helsinki (as revised in 2013). This retrospective study was approved by the Human Research Ethics Committee (No. 18/1367HS). Because of the retrospective nature of the research, the requirement for informed consent was waived.
Open Access Statement: This is an Open Access article distributed in accordance with the Creative Commons Attribution-NonCommercial-NoDerivs 4.0 International License (CC BY-NC-ND 4.0), which permits the non-commercial replication and distribution of the article with the strict proviso that no changes or edits are made and the original work is properly cited (including links to both the formal publication through the relevant DOI and the license). See: https://creativecommons.org/licenses/by-nc-nd/4.0/.
References
- Zayas JO, Feliciano YZ, Hadley CR, et al. Temporal bone trauma and the role of multidetector CT in the emergency department. Radiographics 2011;31:1741-55. [Crossref] [PubMed]
- Little SC, Kesser BW. Radiographic classification of temporal bone fractures: clinical predictability using a new system. Arch Otolaryngol Head Neck Surg 2006;132:1300-4. [Crossref] [PubMed]
- Kennedy TA, Avey GD, Gentry LR. Imaging of temporal bone trauma. Neuroimaging Clin N Am 2014;24:467-86. viii. [Crossref] [PubMed]
- Green L, Wang J, Li C, et al. Management of petrous temporal bone fractures: a 5-year experience from an Australian major trauma centre. Aust J Otolaryngol 2022;5:27. [Crossref]
- Johnson F, Semaan MT, Megerian CA. Temporal bone fracture: evaluation and management in the modern era. Otolaryngol Clin North Am 2008;41:597-618. x. [Crossref] [PubMed]
- Dahiya R, Keller JD, Litofsky NS, et al. Temporal bone fractures: otic capsule sparing versus otic capsule violating clinical and radiographic considerations. J Trauma 1999;47:1079-83. [Crossref] [PubMed]
- Morgan WE, Coker NJ, Jenkins HA. Histopathology of temporal bone fractures: implications for cochlear implantation. Laryngoscope 1994;104:426-32. [Crossref] [PubMed]
- Ishai R, Knoll RM, Chen JX, et al. Otopathologic Changes in the Cochlea following Head Injury without Temporal Bone Fracture. Otolaryngol Head Neck Surg 2018;159:526-34. [Crossref] [PubMed]
- ten Cate WJ, Bachor E. Autoimmune-mediated sympathetic hearing loss: a case report. Otol Neurotol 2005;26:161-5. [Crossref] [PubMed]
- Leigh JR, Moran M, Hollow R, et al. Evidence-based guidelines for recommending cochlear implantation for postlingually deafened adults. Int J Audiol 2016;55:S3-8. [Crossref] [PubMed]
- Battmer R, Pesch J, Stöver T, et al. Elimination of facial nerve stimulation by reimplantation in cochlear implant subjects. Otol Neurotol 2006;27:918-22. [Crossref] [PubMed]
- Cowan B, Oska S, Arianpour K, et al. A Systematic Review of Cochlear Implantation in Temporal Bone Fractures and the Significance of Otic Capsule Involvement. Otol Neurotol 2020;41:1309-15. [Crossref] [PubMed]
- Sun GH, Shoman NM, Samy RN, et al. Do contemporary temporal bone fracture classification systems reflect concurrent intracranial and cervical spine injuries? Laryngoscope 2011;121:929-32. [Crossref] [PubMed]
- Mosnier I, Vanier A, Bonnard D, et al. Long-Term Cognitive Prognosis of Profoundly Deaf Older Adults After Hearing Rehabilitation Using Cochlear Implants. J Am Geriatr Soc 2018;66:1553-61. [Crossref] [PubMed]
- Seidman DA, Chute PM, Parisier S. Temporal bone imaging for cochlear implantation. Laryngoscope 1994;104:562-5. [Crossref] [PubMed]
- Niparko JK, Oviatt DL, Coker NJ, et al. Facial nerve stimulation with cochlear implantation. VA Cooperative Study Group on Cochlear Implantation. Otolaryngol Head Neck Surg 1991;104:826-30. [Crossref] [PubMed]
- Espahbodi M, Sweeney AD, Lennon KJ, et al. Facial nerve stimulation associated with cochlear implant use following temporal bone fractures. Am J Otolaryngol 2015;36:578-82. [Crossref] [PubMed]
- Bigelow DC, Kay DJ, Rafter KO, et al. Facial nerve stimulation from cochlear implants. Am J Otol 1998;19:163-9. [PubMed]
- Eastwood M, Biggs K, Metcalfe C, et al. Outcomes of Cochlear Implantation in Patients with Temporal Bone Trauma: A Systematic Review and Narrative Synthesis. J Int Adv Otol 2021;17:162-74. [Crossref] [PubMed]
- Seyyedi M, Herrmann BS, Eddington DK, et al. The pathologic basis of facial nerve stimulation in otosclerosis and multi-channel cochlear implantation. Otol Neurotol 2013;34:1603-9. [Crossref] [PubMed]
- Matterson AG, O'Leary S, Pinder D, et al. Otosclerosis: selection of ear for cochlear implantation. Otol Neurotol 2007;28:438-46. [Crossref] [PubMed]
- Rayner MG, King T, Djalilian HR, et al. Resolution of facial stimulation in otosclerotic cochlear implants. Otolaryngol Head Neck Surg 2003;129:475-80. [Crossref] [PubMed]
- Shaul C, Dragovic AS, Stringer AK, et al. Scalar localisation of peri-modiolar electrodes and speech perception outcomes. J Laryngol Otol 2018;132:1000-6. [Crossref] [PubMed]
- Trudel M, Côté M, Philippon D, et al. Comparative Impacts of Scala Vestibuli Versus Scala Tympani Cochlear Implantation on Auditory Performances and Programming Parameters in Partially Ossified Cochleae. Otol Neurotol 2018;39:700-6. [Crossref] [PubMed]
- Farinetti A, Ben Gharbia D, Mancini J, et al. Cochlear implant complications in 403 patients: comparative study of adults and children and review of the literature. Eur Ann Otorhinolaryngol Head Neck Dis 2014;131:177-82. [Crossref] [PubMed]
Cite this article as: Morcom S, Hill FCE, Leigh J, Gerard JM. An audit of outcomes of cochlear implantation in patients following temporal bone fracture. Aust J Otolaryngol 2023;6:17.