Environmental responses of virally infected respiratory epithelial cells
Introduction
The human airway epithelium is comprised of ciliated pseudo-stratified airway epithelial cells linked by tight junctions, interspersed with mucous secreting goblet cells and glands (1,2). This structure gives rise to three innate defences against inhaled environmental particles: mucociliary clearance (MCC), barrier function, and the epithelial-derived inflammatory response (2-5).
An inappropriate response to environmental allergens or antigens, or a defective epithelial barrier (allowing otherwise harmless exposures to persistently trigger a mucosal reaction), have been proposed as underlying mechanisms for the airway dysfunction of chronic rhinosinusitis with nasal polyps (CRSwNP) (6-8). Previous experiments have shown elevated expression of Interleukin (IL)-25, IL-33, and thymic stromal lymphopoietin, and altered ciliary morphology and activity in CRSwNP mucosa, indicating that mucosal inflammation and MCC dysfunction may synergistically interact to produce the observed clinical symptomatology and pathology of CRS (7-14).
Environmental allergens, pollutants, and concurrent viral infection may further potentiate innate airway defence dysfunction. Viral infection with superimposed allergens down-regulates the TLR3 mediated anti-viral pathways (2,15), causing enhanced viral replication, cell death, and greater clinical symptomatology, particularly in chronic airway diseases such as asthma (16-18), and is thought to contribute to seasonal variation in CRS exacerbations (19). Likewise, this combination has been shown to alter epithelial inflammation, with a greater release of IL-8, IFNα, and other cytokines (15,20,21). However, there have been conflicting reports of both a deficient (21), or exaggerated (20) IL-6 response. Hence it would be worthwhile to further characterise the effect of rhinovirus infection and common environmental exposures, such as dust mite and pollutants, on both the inflammatory response and other innate defences.
The multi-challenge, air-liquid interface (ALI) model of this study was established to create an in-vitro model of the aetiology of CRSwNP and its differences in responses from normal tissue, allowing for future therapeutic assessments.
Our aims were to evaluate whether: (I) Rhinovirus infected airway epithelial cells respond differently to environmental challenges compared with uninfected cells in ALI cultures; (II) Rhinovirus infected ALI cultures behave differently between cells from healthy sinus mucosa and patients with CRSwNP; and (III) there is synergism between allergen and pollution in virally infected ALI cultures.
Methods
Culturing of human airway epithelium at ALI
Ethical approval for this project was received from the St Vincent’s Hospital Human Research Ethics Committee (LNR/13/SVH/91). Informed consent for the collection of tissue was obtained under the Rhinology Tissue Bank program approved by the St Vincent’s Hospital Human Research Ethics Committee (HREC/10/SVH/117). All laboratory work was performed at the St Vincent’s Centre for Applied Medical Research, and the Woolcock Institute.
Patients included in this study either were undergoing endoscopic surgery for the removal of tumours and had no sinus disease and had nasal mucosa collected, or were CRSwNP patients undergoing endoscopic sinus surgery for treatment, with polyps instead collected as the tissue sample. Patients on systemic or intranasal steroids, macrolide antibiotics, anti-histamines, or other immune-modulating drugs 4 weeks prior to surgery, or with a history of immunological disorders or other sinus diseases were excluded from the study. Tissue collection was carried out during the 2015 calendar year from patients of three ENT surgeons in Sydney who satisfied the inclusion and exclusion criteria.
ALI cultures utilise a semipermeable culture well insert to expose cells to air and thus create an environment more representative of in vivo conditions (Figure 1). These were grown from the harvested tissue as previously described (22). Briefly, tissue samples were rinsed in MEM media (Gibco, USA), carefully dissected to remove adherent bone, cartilage, blood, and mucous extract mucosa, and then cut into small pieces. These were dissociated in MEM media with 1.4 mg/mL of protease isolated from Streptomyces griseus (Sigma-Aldrich, USA) and 10 µg/mL DNAse (Roche Applied Science, Germany) to undergo enzymatic digestion for 1 h at 37 °C, after which 2.5 mL fetal bovine serum (FBS, Gibco, USA) was added to neutralise the enzymes. The supernatant was harvested, centrifuged, resuspended, and seeded in a culture dish for 1h at 37 °C to allow the non-motile non-ciliated cells to adhere to the culture dish. The supernatant containing motile ciliated epithelial cells was then harvested, centrifuged, resuspended, and seeded into a culture flask in 10 mL growth medium [BEBM (Lonza, Switzerland), BEGM SingleQuots (Lonza, Switzerland), 1% penicillin/streptomycin 10K/10K (Lonza, Switzerland), and 0.1% Fungizone antimycotic (Gibco, USA)]. Cells were cultured (37 °C, 5% CO2) with media replacement 24 h later, and subsequently three times a week.
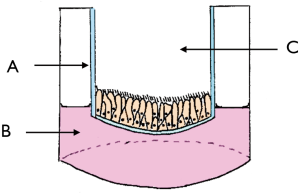
Once 70–90% confluence was reached, cells were detached with 5 mL trypsin (Gibco, USA) (5 min, 37 °C, 5% CO2), then neutralised with 10% FBS in 10 mL MEM (Gibco, USA), resuspended in differentiation medium [1:1 BEBM (Lonza, Switzerland) and DMEM high glucose (Gibco, USA), BEGM SingleQuots (Lonza, Switzerland) without retinoic acid additive, 1% antibiotic/antimycotic (Gibco, USA), 1.5% bovine serum albumin, and 0.03% of 10 mM retinol (Sigma-Aldrich, USA)], and seeded at a density of 5×105 cells/mL in the apical compartment of Transwell inserts coated with type I collagen (Corning, USA), with 600 µL of differentiation medium in the basal compartment (changed every 2 days). When the apical cells were 100% confluent, the apical medium was removed permanently.
Rhinovirus inoculation
Culture wells with visible ciliary differentiation for at least 2 weeks (determined by light microscopy) were selected for experimentation. The basal medium was replaced with steroid-free differentiation medium. Twenty-four hours later the wells were washed three times with phosphate buffered solution (PBS) and incubated (1 h, 37 °C, 5% CO2). Virus-free vehicle or rhinovirus-16 (stock propagated from Ohio HeLa cells) was added to controls and test samples at multiplicity of infection (MOI) =1 (1 h, 37 °C, 5% CO2). The apical suspension was then removed and cells washed three times with PBS to remove remaining virus particles. Fresh basal medium was then added.
Challenge application
Challenges were applied after viral infection to mimic real-life airway responses, and to accommodate for the longer viral replication and response time compared with acute challenge exposure. Challenges were applied to the wells apically in quantities of 200 µL. Diesel particulate matter (DPM) (standard reference material 1650b, National Institute of Standards and Technology, USA) was suspended in steroid-free differentiation medium at a concentration of 100 ng/cm2 using sonication (20 min, 0.5 Hz, 50 amps; UTR200, Hielscher, Germany). House dust mite (HDM) (Stallergenes, Sydney, Australia) was suspended in steroid-free differentiation medium for an exposure of 6,960 AU/cm2. Steroid-free differentiation medium, or “vehicle”, was used as a control challenge. Wells received either vehicle, DPM, HDM, or DPM + HDM combination as challenges. Challenges were evenly distributed amongst all non-infected and infected wells.
Cytotoxicity
Cytotoxic effects of the virus and challenges were assessed using the Tox7 LDH Cytotoxicity Assay (Sigma-Aldrich, USA), by measuring intracellular LDH release following cell lysis. Viral infection did not cause an elevation in LDH release from cultures.
Measuring ciliary beating frequency (CBF)
Wells were visualised using a high-speed camera (Basler 602f; 100 frames/second) attached to a light microscope with 20× magnification and stage heater at 37 °C (Figures 2-4) (Tempcontrol-37, Pecon, Germany), on a 600×600 mm vibration isolation table (ProsciTech, Australia). Video was analysed using the Sisson-Ammons Video Analysis (SAVA) system (Ammons Engineering, USA) (26). SAVA calculated the Gaussian mean frequency for each field after manual filtering to eliminate noise. CBF was measured immediately before challenge application and at 24 h post-challenge. Results are expressed in Hertz (Hz) and fold change from the wells’ pre-challenge baseline to account for baseline variation, and reported as mean ± standard deviation.
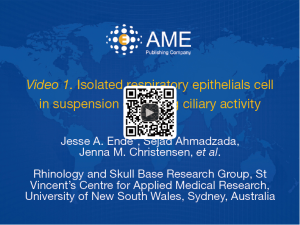
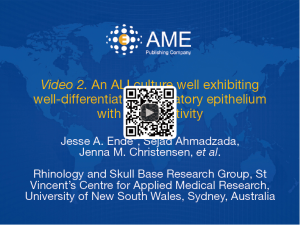
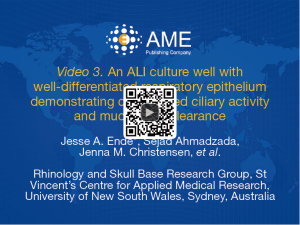
Measuring apical IL-6 release
IL-6 was chosen as a marker of maladaptive airway inflammation following challenge and viral exposure. After 24 h of challenge exposure, the apical media was collected, duplicate supernatants pooled, centrifuged (17,000× g, 20 min), and frozen immediately at −80 °C until use. Apical IL-6 release was analysed using cytometric bead array kits, flow cytometry, and gating strategies (BD Biosciences, USA; FCAP Array software v3) according to the manufacturer’s instructions.
Statistical analysis
Data analysis was performed using SPSS v22 (IBM, USA). Demographic data between healthy and CRSwNP groups was compared using independent t-tests (continuous variables) or Chi squared analysis (categorical variables). Outcomes were compared between infected and non-infected groups, and healthy and CRSwNP subgroups. CBF was determined to be parametric and analysed using paired t-tests for changes from baseline, independent t-tests for comparison of groups, and ANOVA (with Bonferroni correction) to compare challenge responses within each group. Parametric data is reported as the mean ± standard deviation. IL-6 release was represented as fold change from control wells to account for inter-individual variation in IL-6 baseline levels and release. IL-6 release was determined to be non-parametric, and groups were compared using Mann-Whitney U tests and reported as median [interquartile range, (IQR)]. Challenge responses within each group were compared using Kruskal-Wallis one-way analysis of variance tests.
Results
Demographics
A total of 13 patients (age 46.6±12.8, 46% female) had sinonasal mucosa harvested, with 6 (46%) healthy patients and 7 (53%) CRSwNP patients. Baseline demographics are listed in Table 1.
Table 1
Population characteristic | Total | Healthy | CRSwNP | P |
---|---|---|---|---|
N | 13 | 6 | 7 | |
n ALI culture wells | 204 | 96 | 108 | |
Age | 46.6±12.8 | 43.5±12.6 | 49.3±13.2 | 0.440 |
% female | 46% | 50% | 43% | 0.797 |
% asthmatic* | 38% | 17% | 57% | 0.198 |
% dust mite sensitised** | 39% | 50% | 29% | 0.740 |
% smoker* | 0% | 0% | 0% | – |
Time submerged (days) | 9.38±1.26 | 9.83±1.33 | 9.00±1.16 | 0.251 |
Age of culture (days) | 44.3±4.0 | 47.7±2.9 | 41.4±2.2 | 0.001 |
*, three patients (n=1 healthy, n=2 CRSwNP) with missing data. **, n=2 CRSwNP patients with missing data. CRS, chronic rhinosinusitis; ALI, air-liquid interface; CRSwNP, chronic rhinosinusitis with nasal polyps.
A total of 204 ALI wells were cultured, with 96 from healthy and 108 from CRSwNP patients. Half of each patient’s culture was infected with rhinovirus, yielding 102 virally infected and 102 non-infected cultures (infected healthy n=48, infected CRSwNP n=54), of which 26 wells of each received challenges of the control medium, DPM, or HDM, and 24 received the DPM + HDM challenge.
CBF at baseline
At baseline rhinovirus infected wells had a significantly higher CBF compared with uninfected wells (Figure 5A) (11.38±2.38 vs. 10.57±3.07 Hz, P=0.04). While there was no difference between infected and non-infected wells for healthy patients (Figure 5B) (11.94±2.82 vs. 11.51±3.40, P=0.49), there was an increase for CRSwNP patients (10.88±1.78 vs. 9.73±2.49 Hz, P=0.01). Interestingly, CRSwNP wells had a significantly lower CBF than healthy wells for both non-infected and infected groups (Figure 5B) (non-infected: 11.51±3.39 vs. 9.73±2.49 Hz, P<0.01; infected: 10.88±1.78 vs. 11.94±2.82 Hz, P=0.03).
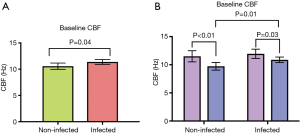
The effect of rhinovirus on the response to challenges
At 24 h post-challenge, viral infection caused a persisting impairment in CBF for all challenges in infected wells compared to baseline (Control: −2.66±1.64 Hz, P<0.001; DPM: −3.28±2.17 Hz, P<0.01; HDM: −1.53±2.24 Hz, P<0.01; DPM + HDM: −0.95±1.99 Hz, P=0.03), while for uninfected cells only vehicle and DPM caused a significant change (Control: −1.53±2.29 Hz, P<0.01; DPM: −1.76±2.72 Hz, P<0.01; HDM: 0.32±2.37 Hz, P=0.50; DPM + HDM 0.71±2.23 Hz, P=0.14). This represented a significantly reduced CBF as a fold change from baseline (Figure 6A) (Control: 0.88±0.21 vs. 0.77±0.14, P=0.03; DPM: 0.88±0.22 vs. 0.73±0.16, P=0.01; HDM: 1.08±0.27 vs. 0.88±0.19, P<0.01; DPM + HDM: 1.11±0.24 vs. 0.93±0.22, P=0.01).
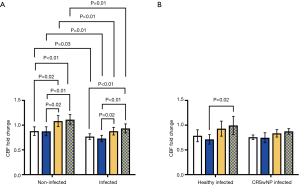
At 24 h post-challenge, apical IL-6 was significantly elevated in virally infected wells for the control, DPM, and HDM challenges (Figure 6A) [Control: 1.00 (0.00) vs. 2.83 (0.34), P<0.01; DPM: 1.20 (0.84) vs. 2.50 (2.52), P=0.02; HDM: 1.02 (0.80) vs. 2.09 (2.33), P<0.01; DPM + HDM: 1.47 (1.02) vs. 2.53 (3.53), P=0.11).
Viral infection and challenges responses in healthy and CRSwNP cultures
At 24 h post-challenge there was no difference in the CBF fold change from baseline of infected healthy and infected CRSwNP wells (Figure 6B) (Control: 0.79±0.19 vs. 0.76±0.08, P=0.61; DPM: 0.71±0.15 vs. 0.75±0.17, P=0.62; HDM: 0.93±0.24 vs. 0.83±0.13, P=0.23; DPM + HDM: 1.00±0.29 vs. 0.87±0.09, P=0.19). Likewise, there was no difference in apical IL-6 release between the groups (Figure 7A) [Control: 1.81 (2.10) vs. 4.46 (2.38), P=0.05; DPM: 1.45 (2.16) vs. 3.32 (1.85), P=0.10; HDM: 1.61 (2.39) vs. 2.73 (2.20), P=0.18; DPM + HDM: 1.25 (2.65) vs. 3.59 (2.50), P=0.18].
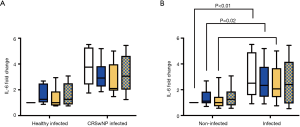
Response to challenge combinations
In both non-infected and infected cells the addition of HDM and HDM + DPM caused a significantly elevated CBF fold change from baseline at 24 h post-challenge (Figure 6A) (non-infected: 0.88±0.21 vs. 0.88±0.22 vs. 1.08±0.27 vs. 1.11±0.24, control vs. DPM P=1.00, control vs. HDM P=0.02, control vs. DPM + HDM P<0.01, DPM vs. HDM P=0.02, DPM vs. DPM + HDM P<0.01, HDM vs. DPM + HDM P=1.00; infected: 0.77±0.14 vs. 0.73±0.16 vs. 0.88±0.19 vs. 0.93±0.22, control vs. DPM P=1.00, control vs. HDM P=0.18, control vs. DPM + HDM P<0.01, DPM vs. HDM P=0.02, DPM vs. DPM + HDM P<0.01, HDM vs. DPM + HDM P=1.00).
However, overall there was no difference in the CBF response between the challenges amongst virally infected cells from both the healthy or CRSwNP groups (Figure 6B).
Although differences exist between infected and non-infected cells, apical IL-6 release did not differ between the challenges within these groups, nor within infected healthy and infected CRSwNP groups (Figure 7).
Discussion
Rhinovirus infection altered the innate defences of the airway epithelial cells cultured at ALI. At baseline, the CBF of all virally infected wells was higher than all non-infected wells (Figure 5A). Interestingly, the CBF at baseline of CRSwNP cells was lower than healthy cells in both the non-infected and infected groups (Figure 5B), despite several weeks of culturing ex vivo prior to experimentation. This could suggest the presence of an intrinsic defect of the CRSwNP cells regarding their MCC function, which may contribute to the disease state in this population. The influence of local microflora had long been removed due to cell washes and antibiotics in culture media, and cell turnover had since occurred several times.
For all challenges, the CBF fold change from baseline remained lower in virally infected cells compared with uninfected cells at 24 h post-challenge. This may indicate an altered chronic adaptation to environmental challenges with concurrent rhinovirus infection, which has been found in previous studies (15,27,28). Additionally, the CBF of infected wells was significantly lower compared with baseline, while for non-infected wells overall there was no difference except with DPM challenge. Together, this implicates rhinovirus infection as being a substantial contributor to an altered environmental epithelial response.
Following challenge exposure, the CBF and IL-6 responses to challenges of infected healthy and infected CRSwNP wells were similar. This suggests that CRSwNP mucosa does not show a deficient innate response in viral infection to these particular challenges compared with healthy sinus mucosa. Previous studies have shown both similar, and differing responses in terms of IL-6 release (29-32), and it is therefore possible that these interactions are complex, with variation also based on the specific type of challenge applied.
With DPM alone failing to produce an epithelial reaction, it is likely HDM was the main contributor to the challenge response. HDM dosing was based on a previous clinical study eliciting nasal symptoms with doses both lower and higher than that used in this experiment (33). This makes it possible that the translation from in vivo to in vitro produced these unexpectedly strong epithelial reactions. Additionally, as this dose of DPM has previously been seen to increase IL-6 release in ALI cultures (34), it is surprising that an effect on cilia was not able to be observed, perhaps owing to insufficient disintegration or dispersion in solution. Together, these results indicate the effects of HDM may have masked the responses to other challenges in terms of ciliary function. Considering that the amount of exposure to an environmental challenge may be important for determining epithelial responses, future experiments could modify these doses to normalise their relative effects and possibly reveal a synergistic interaction. With greater patient numbers, it would be interesting to perform subgroup analysis accounting for clinical sensitisation to HDM, and comparing epithelial responses.
IL-6 release was significantly elevated with rhinovirus infection in all wells, and for infected CRSwNP wells for the challenges of control, DPM, and HDM. This supports previous experimental findings of increased IL-6 with viral infection (30,31). However, with no difference between the challenge responses it appears rhinovirus was the main factor in this result. This disagrees with previous studies suggesting a positive synergistic effect of challenges, and could again be explained by the different types of challenges applied, or the use of submerged cell lines (rather than primary tissue) in some previous experiments (20,35). Additionally, other results have demonstrated impaired IL-6 release in virally infected wells (21). With clinical findings of elevated IL-6 in nasal washes during CRSwNP exacerbations (36), the findings of IL-6 release with viral infection support the role of rhinovirus in contributing to these episodes. However, these conflicting results require further investigation to characterise the inflammation occurring in CRS, viral infection, and challenge exposure concurrently.
Cytotoxicity was primarily assessed to control for its potential confounding effects on cytokine release. While LDH was elevated for the HDM and HDM + DPM challenges, and did not differ from the positive control in these cases, there was no corresponding pattern of IL-6 release, suggesting cell cytotoxicity did not confound the levels of detected cytokines. Cytotoxicity however, is less important for CBF, as this is a function of the beating frequency of the cilia, rather than their absolute numbers.
Primary cultures are more representative of cell behaviour compared with cell lines, which through serial passaging have undergone phenotypic changes (1). As a result of the in vivo physiological conditions of ALI, the cultures in this study more accurately represent the responses of virally infected airway epithelium. However, a single-cell model such as this cannot be used to assess the adaptive immune response in vitro, which may be central to the complex immune response of the airway mucosa.
Conclusions
Rhinovirus infection and CRSwNP are characterised by ciliary dysfunction and maladaptive inflammation. Superimposed environmental challenges likewise contribute to disease burden. This study has demonstrated the impacts of rhinovirus and HDM as potential triggers for airway disease, however true synergism in CBF impairment or IL-6 release was not seen.
Acknowledgments
Dr. Erika Strazdins for her support throughout the entirety of the project.
Funding: None.
Footnote
Conflicts of Interest: RJH serves as unpaid Editor-in-Chief of Australian Journal of Otolaryngology. RJ Harvey is consultant with Medtronic, Olympus, and NeilMed pharmaceuticals. He has been on the speakers’ bureau for Glaxo-Smith-Kline and Arthrocare. J Rimmer has honoraria with Sanofi Aventis, Novartis, Mundipharma, BioCSL, and Stallergenes. The other authors have no conflicts of interest to declare.
Ethical Statement: The authors are accountable for all aspects of the work in ensuring that questions related to the accuracy or integrity of any part of the work are appropriately investigated and resolved. Ethical approval for this project was received from the St Vincent’s Hospital Human Research Ethics Committee (LNR/13/SVH/91). Informed consent for the collection of tissue was obtained under the Rhinology Tissue Bank program approved by the St Vincent’s Hospital Human Research Ethics Committee (HREC/10/SVH/117). The study was conducted in accordance with the Declaration of Helsinki (as revised in 2013).
Open Access Statement: This is an Open Access article distributed in accordance with the Creative Commons Attribution-NonCommercial-NoDerivs 4.0 International License (CC BY-NC-ND 4.0), which permits the non-commercial replication and distribution of the article with the strict proviso that no changes or edits are made and the original work is properly cited (including links to both the formal publication through the relevant DOI and the license). See: https://creativecommons.org/licenses/by-nc-nd/4.0/.
References
- Pezzulo AA, Starner TD, Scheetz TE, et al. The air-liquid interface and use of primary cell cultures are important to recapitulate the transcriptional profile of in vivo airway epithelia. Am J Physiol Lung Cell Mol Physiol 2011;300:L25-31. [PubMed]
- Vareille M, Kieninger E, Edwards MR, et al. The airway epithelium: soldier in the fight against respiratory viruses. Clin Microbiol Rev 2011;24:210-29. [Crossref] [PubMed]
- Matsui H, Randell SH, Peretti SW, et al. Coordinated clearance of periciliary liquid and mucus from airway surfaces. J Clin Invest 1998;102:1125. [Crossref] [PubMed]
- Houtmeyers E, Gosselink R, Gayan‐Ramirez G, et al. Regulation of mucociliary clearance in health and disease. Eur Respir J 1999;13:1177-88. [Crossref] [PubMed]
- Kesimer M, Kirkham S, Pickles RJ, et al. Tracheobronchial air-liquid interface cell culture: a model for innate mucosal defense of the upper airways? Am J Physiol Lung Cell Mol Physiol 2009;296:L92-100. [PubMed]
- Tan BK, Schleimer RP, Kern RC. Perspectives on the etiology of chronic rhinosinusitis. Curr Opin Otolaryngol Head Neck Surg 2010;18:21. [Crossref] [PubMed]
- Fokkens WJ, Lund VJ, Mullol J, et al. EPOS 2012: European position paper on rhinosinusitis and nasal polyps 2012. A summary for otorhinolaryngologists. Rhinology 2012;50:1-12. [Crossref] [PubMed]
- Chin D, Harvey RJ. Nasal polyposis: an inflammatory condition requiring effective anti-inflammatory treatment. Curr Opin Otolaryngol Head Neck Surg 2013;21:23-30. [Crossref] [PubMed]
- Toskala E, Nuutinen J, Rautiainen M. Scanning electron microscopy findings of human respiratory cilia in chronic sinusitis and in recurrent respiratory infections. J Laryngol Otol 1995;109:509-14. [Crossref] [PubMed]
- Allakhverdi Z, Comeau MR, Jessup HK, et al. Thymic stromal lymphopoietin is released by human epithelial cells in response to microbes, trauma, or inflammation and potently activates mast cells. J Exp Med 2007;204:253-8. [Crossref] [PubMed]
- Reh DD, Wang Y, Ramanathan M Jr, et al. Treatment-recalcitrant chronic rhinosinusitis with polyps is associated with altered epithelial cell expression of interleukin-33. Am J Rhinol Allergy 2010;24:105. [Crossref] [PubMed]
- Lai Y, Chen B, Shi J, et al. Inflammation-mediated upregulation of centrosomal protein 110, a negative modulator of ciliogenesis, in patients with chronic rhinosinusitis. J Allergy Clin Immunol 2011;128:1207-15.e1. [Crossref] [PubMed]
- Li YY, Li CW, Chao SS, et al. Impairment of cilia architecture and ciliogenesis in hyperplastic nasal epithelium from nasal polyps. J Allergy Clin Immunol 2014;134:1282-92. [Crossref] [PubMed]
- Lam M, Hull L, Imrie A, et al. Interleukin-25 and interleukin-33 as mediators of eosinophilic inflammation in chronic rhinosinusitis. Am J Rhinol Allergy 2015;29:175-81. [Crossref] [PubMed]
- Golebski K, Luiten S, van Egmond D, et al. High degree of overlap between responses to a virus and to the house dust mite allergen in airway epithelial cells. PloS one 2014;9:e87768. [Crossref] [PubMed]
- Message SD, Laza-Stanca V, Mallia P, et al. Rhinovirus-induced lower respiratory illness is increased in asthma and related to virus load and Th1/2 cytokine and IL-10 production. Proc Natl Acad Sci U S A 2008;105:13562-7. [Crossref] [PubMed]
- Hackett TL, Singhera GK, Shaheen F, et al. Intrinsic phenotypic differences of asthmatic epithelium and its inflammatory responses to respiratory syncytial virus and air pollution. Am J Respir Cell Mol Biol 2011;45:1090-100. [Crossref] [PubMed]
- Gavala ML, Bashir H, Gern JE. Virus/allergen interactions in asthma. Curr Allergy Asthma Rep 2013;13:298-307. [Crossref] [PubMed]
- Lima JT, Paula FE, Proença-Modena JL, et al. The seasonality of respiratory viruses in patients with chronic rhinosinusitis. Am J Rhinol Allergy 2015;29:19-22. [Crossref] [PubMed]
- Bossios A, Gourgiotis D, Skevaki C, et al. Rhinovirus infection and house dust mite exposure synergize in inducing bronchial epithelial cell interleukin‐8 release. Clin Exp Allergy 2008;38:1615-26. [Crossref] [PubMed]
- Xatzipsalti M, Psarros F, Konstantinou G, et al. Modulation of the epithelial inflammatory response to rhinovirus in an atopic environment. Clin Exp Allergy 2008;38:466-72. [Crossref] [PubMed]
- Kim JH, Rimmer J, Mrad N, et al. Betadine has a ciliotoxic effect on ciliated human respiratory cells. J Laryngol Otol 2015;129:S45-50. [Crossref] [PubMed]
- Ende JA, Ahmadzada S, Christensen JM, et al. Isolated respiratory epithelials cell in suspension exhibiting ciliary activity. Asvide 2019;6:062. Available online: http://www.asvide.com/article/view/30439
- Ende JA, Ahmadzada S, Christensen JM, et al. An ALI culture well exhibiting well-differentiated respiratory epithelium with ciliary activity. Asvide 2019;6:063. Available online: http://www.asvide.com/article/view/30440
- Ende JA, Ahmadzada S, Christensen JM, et al. An ALI culture well with well-differentiated respiratory epithelium demonstrating coordinated ciliary activity and mucociliary clearance. Asvide 2019;6:064. Available online: http://www.asvide.com/article/view/30441
- Sisson JH, Stoner J, Ammons B, et al. All‐digital image capture and whole‐field analysis of ciliary beat frequency. J Microsc 2003;211:103-11. [Crossref] [PubMed]
- Yeo NK, Hwang YJ, Kim ST, et al. Asian sand dust enhances rhinovirus-induced cytokine secretion and viral replication in human nasal epithelial cells. Inhal Toxicol 2010;22:1038-45. [Crossref] [PubMed]
- Eddleston J, Lee RU, Doerner AM, et al. Cigarette smoke decreases innate responses of epithelial cells to rhinovirus infection. Am J Respir Cell Mol Biol 2011;44:118-26. [Crossref] [PubMed]
- Wang JH, Kwon HJ, Chung YS, et al. Infection rate and virus-induced cytokine secretion in experimental rhinovirus infection in mucosal organ culture: comparison between specimens from patients with chronic rhinosinusitis with nasal polyps and those from normal subjects. Arch Otolaryngol Head Neck Surg 2008;134:424-7. [Crossref] [PubMed]
- Peters AT, Kato A, Zhang N, et al. Evidence for altered activity of the IL-6 pathway in chronic rhinosinusitis with nasal polyps. J Allergy Clin Immunol 2010;125:397-403.e10. [Crossref] [PubMed]
- Kim JH, Kim YS, Cho GS, et al. Human Rhinovirus-induced Proinflammatory Cytokine and Interferon-β Responses in Nasal Epithelial Cells From Chronic Rhinosinusitis Patients. Allergy Asthma Immunol Res 2015;7:489-96. [Crossref] [PubMed]
- Contoli M, Ito K, Padovani A, et al. Th2 cytokines impair innate immune responses to rhinovirus in respiratory epithelial cells. Allergy 2015;70:910-20. [Crossref] [PubMed]
- Oldenbeuving NB, Kleinjan A, Mulder P, et al. Evaluation of an intranasal house dust mite provocation model as a tool in clinical research. Allergy 2005;60:751-9. [Crossref] [PubMed]
- Auger F, Gendron MC, Chamot C, et al. Responses of well-differentiated nasal epithelial cells exposed to particles: role of the epithelium in airway inflammation. Toxicol Appl Pharmacol 2006;215:285-94. [Crossref] [PubMed]
- Shin SH, Ye MK, Jeon BG, et al. Cooperative effect of Alternaria and rhinovirus on the activation of nasal polyp epithelial cells. J Rhinol 2012;19:112-8.
- Rank MA, Hagan JB, Samant SA, et al. A proposed model to study immunologic changes during chronic rhinosinusitis exacerbations: data from a pilot study. Am J Rhinol Allergy 2013;27:98. [Crossref] [PubMed]
Cite this article as: Ende JA, Ahmadzada S, Christensen JM, Oliver B, Estephan T, Alvarado R, Rimmer J, Harvey RJ. Environmental responses of virally infected respiratory epithelial cells. Aust J Otolaryngol 2019;2:8.